Can Performance for Food Stuff
Can Performance for Food Stuff
Tinplate’s long and successful record as a packaging material is due to its consistent performance in preserving packaged goods so that they are intact and still in good condition when the container is opened. The favourable properties of tinplate are important in this connection, but just as important is the research and development work which goes into understanding the interactions which can take place between the container and its contents and into tailoring the properties of the packaging material so as to overcome possible corrosion problems. In order to understand the can/product system, it is useful first to look briefly at the canning process.
Canning of foodstuffs
The purpose of canning foodstuffs is to preserve the freshness of the product. Close co-operation is maintained between farms and canneries and the latter are usually situated in the principal farming areas. Once fruit or vegetables are picked, care is taken to see that the food is packed within a few hours whilst in the peak of condition. It is interesting to note that whereas “canned” foods are commonly contrasted with “fresh foods”, in fact the contents of the can are often hygienically and nutritionally superior to fresh foodstuffs which have been on display for a day or so at a retail outlet.
The principal foodstuffs, which are canned, comprise: fish, fruit, meat, milk products, pet foods, soups and vegetables. At the cannery the food is inspected, cleaned and graded; when necessary it is prepared by peeling, shelling, cutting up, etc. In some cases the food is given a short cook so that any initial shrinkage takes place before the can is filled. As will be seen later, the amount of air in the headspace must be kept as low as possible, to avoid corrosion problems and after filling, the can closure is seamed on. The vital step in the canning of foodstuffs is that of sterilisation. The filled and sealed cans are passed through a processing unit in which they reach the required temperature to kill bacteria which could be harmful to health, or cause spoilage of the contents. The contents are also cooked during this processing so that they require little or no further preparation. The slight vacuum in the can prior to this heating helps to avoid expansion of the container during processing and the cans are rapidly cooled after the heating stage.
In general, meat, fish and soups in cans may be stored for some years without any deterioration in quality. In the case of beverages, these are pasteurised by heating the can, and a sterilisation cooking process is not required.
The container/product system
Food products and beverages are all extremely complex systems, which can differ with respect to pH, buffering properties, content of corrosion inhibitors or accelerators, and consistency of product. Foodstuffs may be broadly classified into categories such as “highly corrosive”, “moderately corrosive”, “slightly corrosive”, “highly detinning” etc., on account of their typical behaviour. However, a number of variables may intervene to alter the pattern of behaviour. These factors include: biological variance in the natural product; trace residues from exterior influences such as heavy use of fertilizer, use of pesticides, feeding; processing effects, e.g. pre-treatment with sulphur dioxide; and specific filling conditions which influence the amount of oxygen in the headspace of the can.
Can performance
Optimum can performance depends on controlling the electrochemical reactions, which tend to occur within the can/product system. Some effects, which may appear, are general type corrosion, which proceeds gradually and tends to spread laterally rather than penetrating metal at localised points. It can be controlled within acceptable quality limits by good canning, storage and distribution practices. Pitting type corrosion occurs at sharply localised areas and tends to perforate the metal with very little visible evidence and without noticeable effect on product appearance, although it may eventually result in leakage and
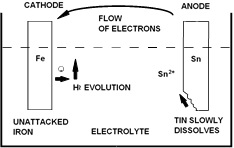
e corr- i bacteriological spoilage of the can contents. It can be avoided by proper matching of product and can material systems.
To visualise the corrosion processes which may occur in a filled can, it is helpful to consider a simple model (Figure Left). In the case shown, when tin and iron electrodes are immersed in
an electrolyte, each will develop an uncoupled potential, which
(Schematic representation of electrochemical processes taking place in a tinplate can)
can be measured by suitable means. In connecting the two electrodes, electrons will flow through the circuit and polarisation will take place at the electrodes. At equilibrium the electron flow will constitute the corrosion current icorr. At the tin electrode tin will pass into solution slowly as ions and electrons will be released to electrolyte at the iron electrode resulting in evolution of molecular hydrogen. One would expect iron to be anodic to tin and in fact in solutions of mineral salts or in the atmosphere, this is the case. Fortunately, in the presence of many natural products and with limited oxygen supply in the food can, the reverse is the case. This is because the complexes which tin forms with carboxylic acids reduce the concentration of free tin ions to low levels, and the self-corrosion of tin in the absence of cathode depolarisers is very small, due to its high hydrogen overpotential.
Therefore, in unlacquered cans, when steel is exposed to the can contents (at pores or scratches), in the absence of oxygen, tin dissolves preferentially. In view of the relatively large area of tin compared to exposed iron, this tin dissolution occurs smoothly and slowly; the presence of dissolved tin also inhibits the cathodic reaction on exposed steel and the net effect is a long period of an almost steady corrosion rate lasting until substantial areas of steel are exposed and iron begins to pass into solution. Hydrogen evolved at a slow rate in the initial period of corrosion seems to pass into, or through, the steel but at the later, faster, stages of corrosion, considerable quantities of hydrogen are produced, which will eventually distort the shape of the can. Such “hydrogen swells” are considered unacceptable since they cannot be distinguished visually from those which would arise from bacterial activity in the can, and such damaged containers must be discarded. However, due to the protective action of the tin in a largely oxygen-free environment and since in many natural products tin complexes are formed which inhibit corrosion, hydrogen swells normally occur only after a longer period than the expected shelf life of the product at the point of sale.
In some foods, the difference in corrosion potential between tin and steel may be small; coupling tin and steel together in such a foodstuff alters the steel potential but not sufficiently to provide complete cathodic protection. Thus, iron exposed at pores may dissolve and the cans fail by perforation. The formation of films on tinplate, for example by reaction with sulphur-bearing compounds, may also result in pitting; film formation hinders the anodic reaction on tin and hence its ability to protect exposed steel.
An important influence on the rate of dissolution of tin is the presence of cathode depolarisers such as oxygen, nitrate or sulphur dioxide, which permit an alternative cathodic reaction to the reduction of hydrogen ions to hydrogen. The presence of dissolved oxygen in the electrolyte thus greatly increases the corrosion rate of tinplate. Oxygen reduction to hydroxyl ions proceeds rapidly on tin, the rate of the reaction being frequently governed by mass transport rather than electrochemical influences. Oxygen is initially present in the can headspace and this leads to an initial period of tin dissolution after which the rate slows down when all the oxygen is consumed. Elimination of oxygen prior to can closure thus greatly contributes to shelf life. Other depolarisers, which can accelerate the corrosion rate, are nitrates, colouring matter in dark fruits, copper traces and so on. Nitrates, which may arise from fertiliser residues, have been particularly troublesome on occasion. Some compounds, such as sugars and starch products are believed to act as corrosion inhibitors.
So far, only the simple system: steel with a tin coating, has been considered but in practice, tinplate is a much more complex system, as can be seen from Figure below which, in section, illustrates the various layers present on the steel surface in a commercial tinplate.
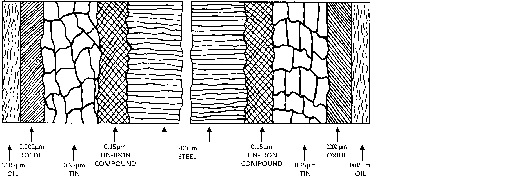
- Figure - Layers existing on the steel substrate in commercial tinplate
The iron-tin intermetallic compound which is formed by reaction between the tin coating and the steel substrate, largely during flow melting, plays an important part in determining the corrosion resistance of the tinplate. As will be described later, the alloy-tin couple (ATC) test is important as an indicator of alloy layer continuity in a citrus pack application, a factor related to shelf life. Once a considerable amount of tin has dissolved, this alloy layer constitutes a second line of defence for the underlying steel and also slows down dissolution of tin by reducing the iron cathode area.
Depending on the type and intensity of the electro-chemical treatment, the passivation film on tinplate consists of tin oxides and various amounts of chromium(III) oxide and metallic chromium. Treatments can be adopted so as to produce various film compositions to meet particular end-use requirements, but in practice only a few specific treatments are applied.
Protecting can contents
Whereas protecting the integrity of the container is an essential requirement, no less important is the need to ensure that the can contents are protected from bacteriological attack and from contamination by traces of harmful substances. It is important, when tinplate containers are opened by the consumer, that they show no changes in appearance internally or in the character of the can contents. Even if certain changes are not associated with harmful effects, nevertheless they are not acceptable if they suggest deterioration to the consumer. There must be no uncharacteristic changes in flavour, colour or texture of the foodstuff and the pack must conform with regulations regarding metal content of the packed product.
As has been shown, the electrochemical processes taking place within a can often involve the dissolution of some tin. It is well established that no ill effects can be attributed to regular long term ingestion of tin at the levels normally encountered with good canning practice. A few instances have been reported of temporary irritation of the gastro-intestinal tract caused by ingestion of products with abnormally high tin contents. However, once the tin was voided, no long term harmful effects were experienced. It should be emphasised that only tin in solution appears to cause these temporary upsets at high levels; in solid foods, most of the tin appears to be combined with food constituents such as proteins and is rendered innocuous. In many countries, a limit of 250 ppm tin has been accepted for many years as a working recommendation for tin in foodstuffs. In the UK, a tin in food legislation was published in 1992, setting the limit for the level of tin in food at 200ppm. It has been suggested that lower limits might be applied to some special categories of canned products, for example baby foods and fruit juices. Extremely small amounts of tin may cause some loss of clarity in beer and the flavour of this product is extremely sensitive to trace metal content, so that multiple lacquer coatings are used for the interior of such cans.
Restrictions on the metal content of foodstuffs are under constant review, either as legislation or as recommendations which have some legal significance. National and international standards are continually being revised and changes in permitted levels are made from time to time.
Sulphur-containing compounds, including proteins and allied substances, occur naturally in most animal and vegetable products. During heat processing, these can break down to give free sulphide and hydrosulphide ions and evolution of hydrogen sulphide. Serious discolouration may occur on unpassivated tinplate or at scratches on passivated surfaces. Hydrogen sulphide in the headspace may, at pH less than 6, cause formation of iron sulphide, which may detach so as to discolour the contents of the can. Lacquering should overcome the problem of sulphide staining; for products known to produce this effect strongly, internal lacquers pigmented with zinc oxide may be used. These have only a slight pearly appearance and the zinc sulphide which is formed, is similarly a white product, so that no colour change occurs. It should be noted that sulphide staining affects only the appearance of the can interior and has no actual harmful effect on the foodstuff.
When considering grades of tinplate for packing particular products, it is possible only to provide general guidelines. Natural products vary in their corrosive effect with place of origin, with season, and with harvesting and other processes that precede canning. Other factors, which play a part, include canning technology, period of storage and transport of filled cans and requirements for can performance as influenced by national marketing conditions or legislation.
- Source: The International Tin Association (formerly ITRI Ltd)